Research
We currently have four research projects in our group. In two experiments we explore quantum metrology with ensembles of ultracold atoms and atomic vapor cells. The other two experiments investigate quantum interfaces between atoms and solid-state nanosystems such as semiconductor quantum dots and nanomechanical oscillators. We closely collaborate with other research groups in Basel, at other Swiss universities and abroad. Our activities are integrated in the NCCR Quantum Science and Technology (QSIT) and the Swiss Nanoscience Institute (SNI) and receive funding from the European Research Council (ERC) and the Quantum Flagship.
Current research projects
Entanglement and quantum metrology on atom chips
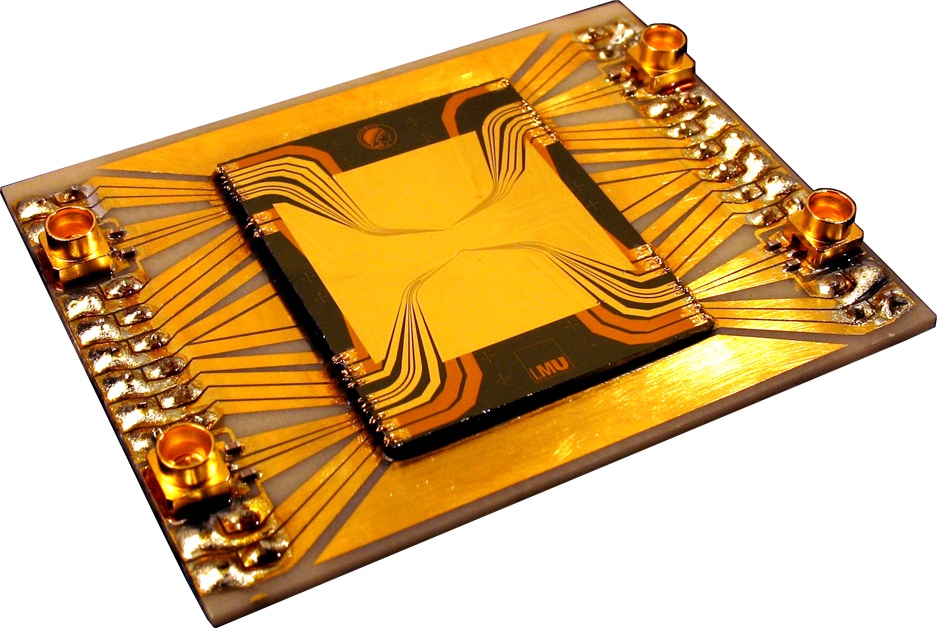
Entanglement is a fundamental concept of quantum physics that still presents many conceptual challenges, in particular when applied to many-body systems of indistinguishable particles. At the same time, it is an essential resource for quantum technologies such as quantum metrology with atomic clocks and interferometers. In this project we use small atomic Bose-Einstein condensates on an atom chip - an exceptionally well-controlled quantum many-body system - to study many-particle entanglement and harness it for quantum metrology with chip-based interferometers.
Key results obtained so far include the creation of spin-squeezed states, a chip-based atom interferometer operating beyond the standard quantum limit, the observation of Bell correlations and Einstein-Podolsky-Rosen steering in a Bose-Einstein condensate.
Hybrid optomechanics with atoms and membranes
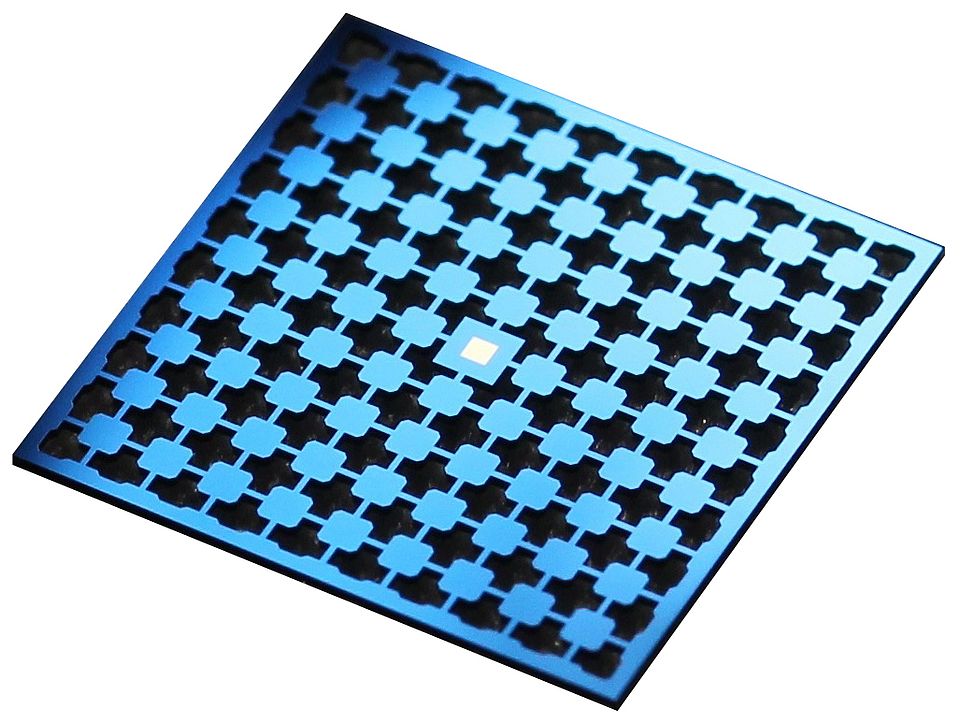
Atoms are routinely prepared and manipulated in the quantum regime using the powerful techniques of laser cooling and trapping. To achieve similar control over the vibrations of nanofabricated mechanical oscillators is a goal that is vigorously pursued. In this project, we explore the new possibilities offered by hybrid mechanical-atomic systems. We use laser light to generate a long-distance coupling between an ultracold atomic ensemble in a trap and a nanomechanical membrane oscillator in an optical cavity. Besides the intriguing perspective of observing quantum phenomena in engineered mechanical devices that are visible to the bare eye, such systems open up new avenues for quantum measurement of mechanical vibrations with potential impact on mechanical quantum sensors and transducers for accelerations, forces and fields.
Research highlights include the observation of strong coupling between atomic spins and a mechanical oscillator, the engineering of different Hamiltonian and dissipative interactions in this hybrid quantum system, and the demonstration of coherent feedback cooling of a mechanical oscillator with the atoms.
Quantum memories for single photons
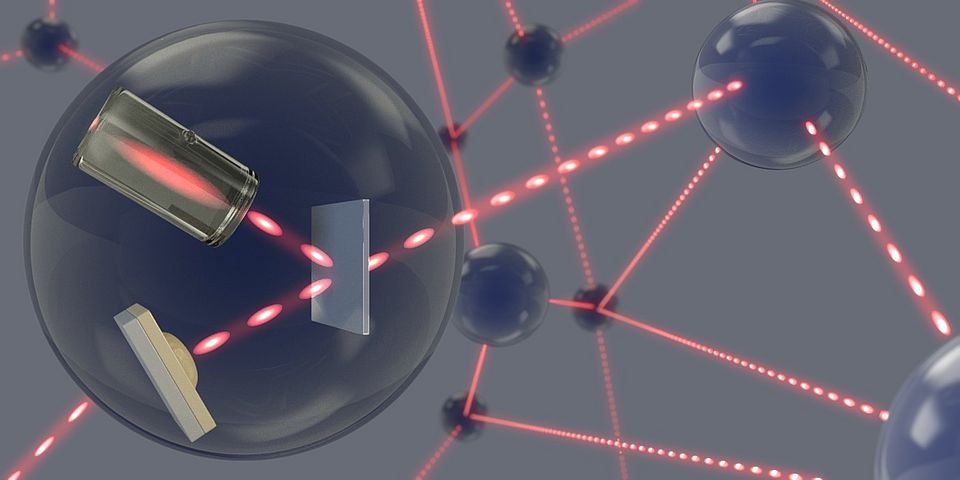
Semiconductor quantum dots are excellent single-photon sources, providing triggered single-photon emission at a high rate and with high spectral purity. Independently, atomic ensembles have emerged as one of the best quantum memories for single photons, providing high efficiency storage and long memory lifetimes. In this project, we aim at combining these systems for the first time, using a new type of quantum dot that emits photons at a wavelength compatible with Rubidium atoms, and a Rubidium quantum memory that is sufficiently broadband to accept these photons. The memory will be used to store and retrieve quantum dot single photons, realizing a quantum interface between these disparate physical systems. This will form the basis for experiments on hybrid entanglement and quantum networks.
This project is a close collaboration with the Warburton group (also at University of Basel). So far, we observed transform-limited single photons from GaAs quantum dots at the wavelength of the rubidium D2-line and demonstrated tuning of their temporal shape. Moreover, we implemented a broadband quantum memory in hot rubidium vapour and used it to store and retrieve single photons from a downconversion source.
Microwave field imaging
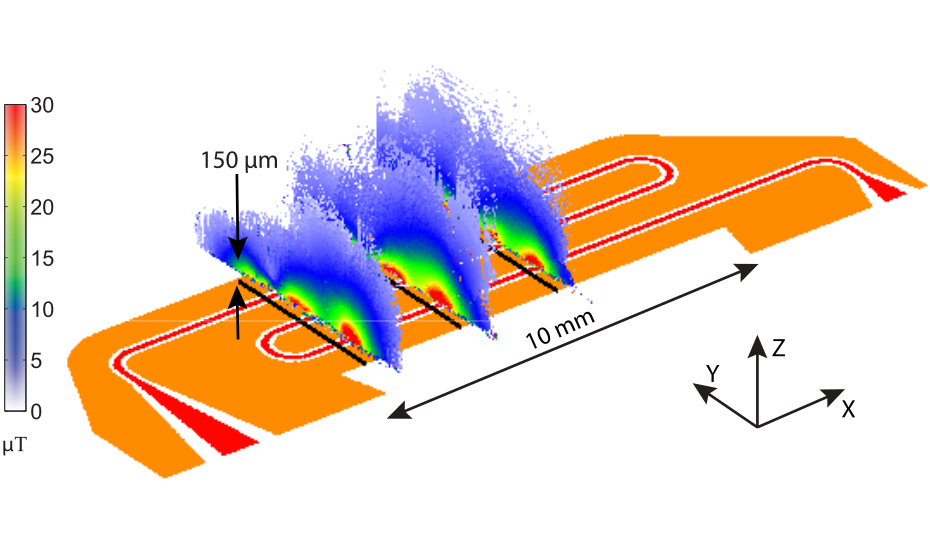
Integrated microwave circuits are an essential element of modern communication technology. For function and failure analysis of such devices, a well-calibrated method for microwave field imaging is needed. In this project, we use atoms for high-resolution imaging of microwave fields near integrated circuits. By recording Rabi oscillations on microwave transitions with a camera, we obtain an intrinsically calibrated image of the microwave field distribution. Further applications of our technique include the characterization of atomic clocks, the study of atomic diffusion and atom-wall collisions in vapor cells, and the operation as an atomic spectrum analyzer.
We have successfully demonstrated our technique with hot rubidium vapor in microfabricated vapor cells and are developing it further for applications in industry.